The translation and labelling of illuminating passages from the following article outline, in my opinion, the essence of the problem of fatty-degenerative osteonecrosis in the jawbone (FDOJ): the misdirected adipogenesis in the bone marrow of the jaw as a prerequisite for overexpressed RANTES/CCL5 signalling. I would like to make this illuminating investigation available to ICOSIM members; the essential passages are marked.
Sincerely,
J. Lechner
Defining osteoblast and adipocyte lineages in the bone marrow
J.L. Pierce3, D.L. Begunb, J.J. Westendorf*’’1, M.E. McGee-Lawrencea,d‘”
** Department of Cellular Biology and Anatomy, Medical College of Georgia, Augusta University, Augustat GA, USA b Department of Orthopedic Surgery, Mayo Clinic, Rochester, MN, USA c Department of Biochemistry and Molecular Biology> Mayo Clinic, Rochester, MN, USA d Department of Orthopaedic Surgery, Augusta University, Augusta, GA, USA
Corresponding author:
E-mail address: mmcgeelawrence@augusta.edu (M.E. McGee-Lawrence).
https://doi.Org/10.1016/j.bone.2018.05.019
Received 16 March 2018; Received in revised form 16 May 2018; Accepted 16 May 2018 8756-3282/ © 2018 Elsevier Inc. All rights reserved.
- The micro-environment of the bone marrow is heterogeneous
Bone is a metabolically active tissue with complex physiological functions. Bone marrow is a semi-fluid component of bone that occupies the endosteal space. It consists of a diverse population of cell types, including those involved in bone development, haematopoiesis, tissue repair and endocrine regulation. Many of the mature cell types present in bone marrow stem from common precursors. One multipotent precursor, the bone marrow mesenchymal stem/stromal cell (BMSC), comprises a heterogeneous population of stem cells which can mature into chondrogenic, myogenic, osteogenic or adipogenic cells on receipt of the appropriate signal [1]. BMSCs retain their stem-like nature throughout their development, until they are differentiated by stressors or physiological needs. During BMSC differentiation, changes occur in the transcription profile, cellular metabolism and morphology, consistent with the cell lineage committed to. With age, and under pathological conditions, the normal balance between osteogenic and adipogenic cell populations can shift towards the latter, threatening bone health and integrity [2]. Here we look at the osteoblast-adipocyte relationship in the bone marrow, and shed light on what these two cell types can look like. Advances in this area could lead to new insights into prevention of age- and pathology-related fractures and metabolic syndromes. - BMSC commitment to differentiation of osteoblasts or adipocytes
BMSCs are the multipotent precursors responsible for maintaining the non-hematopoietic cell population in the bone and bone marrow. The commitment of BMSCs to osteogenesis is a strictly regulated process which depends on a variety of signalling mechanisms during development and in adulthood (Fig. 1). Early regulators of osteogenesis in the BMSC population include Wnt/β-catenin signalling and bone morphogenic proteins(BMPs), hedgehog proteins(i.e. Sonic Hedgehog, Indian Hedgehog), endocrine hormones such as parathyroid hormone (PTH), epigenetic regulators and various growth factors [3-5], the major osteoblast transcription factors – transcription factor 2 (Runx2) and Osterix 1 (Osx1/Sp7) – play a key role in the induction of osteogenetic differentiation and shift the gene expression profile of BMSC to osteogenic genes which control an extracellular matrix (ECM) of type 1 collagen deposition [6]. BMSCs dedicated to osteogenesis further develop the genetic profile and morphology of the osteoblast and express genes such as alkaline phosphatase, osteoprotegerin, type 1 collagen, and later osteocalcin, as these cells shift towards a terminal osteocyte morphology [7]. In adults, the main task of BMSC-derived osteoblasts is to produce and secrete osteoid and mineralisation factors that are associated with the activity of osteoclasts (which resorb the bone matrix) to reshape the bone in accordance with metabolic and structural requirements of the body. In cases where BMSC is not committed to osteogenesis, bone formation may become decoupled from resorption, decreasing bone density and tissue integrity [8]. Fig. 1. Differentiation of BMSCs into osteogenic and adipogenic lineages. Bone marrow mesenchyme stem cells (BMSCs) are differentiated into osteoblasts by transcription factors such as Runx2 and Osxl or by adipocytes via PPAR-γ and C/EBPa. Osteoblasts derived from BMSC may further differentiate into mature osteocytes or become lipid-storing cell types. This diagram illustrates a possible mechanism of osteoblast-adipocyte rearrangement and reduction of lipid storage in osteogenic cells via histone deacetylase 3 (Hdac3) and its associated cofactors.
AdipocytesUnder certain conditions, BMSCs may alternatively be directed to adipogenesis. In fact, some regard the differentiation of BMSC into the adipocyte line as the ‘standard’ path [9]. The differentiation of BMSC into bone marrow adipocyte involves the expression of the major transcription factors: Peroxisome proliferator-activated receptor gamma (PPAR-γ) and CCAAT/enhancer-binding protein a (C/EBPa) [10]. Bone marrow adipocytes characteristically store cytoplasmic lipid droplets and express a number of genes related to lipid storage (i.e. Cidec, Plinl), fatty acid metabolism (i.e. Fasn, lipases such as ATGL and HSL) and adipocyte function (i.e. adiponectin, LepR) [11]. While the role of bone marrow adipocytes is still unknown, there is evidence that these cells may serve as an energy store for the bone[12]. In addition, marrow adipocytes interact with other bone cells in various recesses where they may regulate bone remodelling, repair and gland regulation processes [13]. These lipid-storing cells are therefore an important part of skeletal biology and may offer new insights into the activity of osteoblasts and other BMSC-derived cell types.

- Cellular energetics of BMSC-derived osteoblasts and adipocytes
In a similar way that different tissues vary in their energy needs due to their physiological functions, during commitment to terminal differentiation, the cellular energetic profile of BMSCs changes (Fig. 2). The mesenchymal progenitor cells rely mainly on glycolysis for energy metabolism, while the energy requirement of these cells increases dramatically during osteogenesis [14]. Importantly, glucose metabolism in BMSCs is increased due to unregulated glutamine transporter expression, which precedes the expression of Runx2 within differentiating pre-osteoblasts, providing the energy required to produce the type 1 collagen that comprises the ECM [15]. Mature osteoblasts also require a high ATP yield from fuel sources to produce and secrete significant quantities of osteoids, and therefore fatty acid oxidation in addition to glucose metabolism to meet this energy requirement [16]. While the energetics of late-stage osteogenic cells are sufficient for their function, the use of oxidative phosphorylation results in high energy. The yield can contribute to the production of excess reactive oxygen species which may cause cell damage over time.
As a rule, bone marrow adipocytes have differing metabolic needs from osteogenic cell types, which is also reflected in their cellular energetics. Instead of using glucose metabolism to produce energy, these lipid-storing cells are characterised by their fatty acid metabolism and are highly dependent on the lipolysis of their intracellular lipid stores to make free fatty acids (FFA) available for metabolism via oxidative phosphorylation [17]. Thanks to their use of fatty acids as intracellular lipid droplets, marrow adipocytes can serve as important reservoirs of energy when other sources (e.g. glucose) are exhausted. However, an excess of FFA produced by the metabolism of lipid droplets may have lipotoxic effects in BMSCs and osteoblasts by interrupting cell function and inducing cell death [18]. The harmful effects of this fatty acid metabolism on osteoblasts can be prevented by inhibiting fatty acid synthesis [19]. The fascinating relationship between fat-storing and bone-forming cells involves crosstalk at a metabolic level. The delicate balance between glycolysis and oxidative phosphorylation – particularly of lipid metabolytes – in osteogenic and adipogenic cell types is an active area of interest that may offer new insights into the maintenance of skeletal health.4. Lipid storage in non-adipogenic skeletal cells
While it is clear that both osteoblast precursors and adipocytes are found in bone marrow, their identity can be difficult to detect, since lipid accumulation is often a defining feature of adipocytes rather than line-defining transcription factors. One of the challenges in histology-based study of bone marrow adiposis is that the lipid droplets in bone marrow cells take up a large part of the cell volume, leaving little room for studying other organelles, including the nucleus, where line-directed transcription factors are typically found. However, the biochemical and metabolic pathways which produce and dissolve lipid droplets are the same in all cells and not explicit for adipocytes. Storing intracellular lipid droplets in non-adipogenic line cells is not a novel concept. For example, lipid storage in liver hepatocytes as a result of increased alcohol consumption has been studied extensively. In contrast, intracellular lipid storage in osteoblast lines is not currently widely recognised by bone experts, despite the increasing evidence for the existence of this mechanism. Chronic alcohol consumption results in increased intracellular lipid deposition in osteocytes, which often precedes osteocyte apoptosis and disease-related osteonecrosis [20]. It has also been shown that chondrocytes contain intracellular lipids, and that their frequency increases with age [21].Fig. 2. Displacement of cellular energy profiles by BMSC differentiation. The precursor BMSC population is highly glycolytic and depends on glucose for its energy metabolism. Since BMSCs are committed to osteogenesis, the energy requirements of osteoid production require metabolic processes that produce more ATP – e.g. the metabolism of fatty acids for oxidative phosphorylation. Older osteoblasts use a combination of glycolysis and oxidative phosphorylation to meet their energy needs. However, this balance must be strictly regulated to reduce the formation of reactive oxygen species (ROS) and subsequent cell damage. In contrast to mature BMSCs, adipocytes produce a large portion of their ATP by fatty acid oxidation and oxidative phosphorylation. The metabolic profile of lipid-positive pre-osteoblasts is still unknown, but the intermediate morphology of this cell type suggests that in times of high energy requirement it can store both energetic processes and lipid droplets for fatty acid metabolism.
Although lipid storage in osteoblasts was considered potentially harmful in the above-mentioned studies, it was also suggested as a crucial step in osteogenesis. Using Triascin C to impede lipid droplet formation in BMSCs caused a remarkable decrease in differentiation of osteoblasts as measured by alkaline phosphatase and von Kossa staining [43]. However, while fat accumulation in osteoblasts is often associated with pathological conditions, the physiological roles of these lipid-storing cells remains unclear and may vary according to the stage of osteoblastic differentiation.
Despite the possibility that lipid droplets support the function of osteoblasts, it is important to note that storage of excess bone marrow fat may adversely affect bone density and tissue health if lipid deposition exceeds the energy required for differentiating BMSCs. Adipose tissue in the metaphyseal region of the bone marrow cavity tends to occupy a space that might otherwise hold trabecular bone, and there is increasing evidence that bone marrow fat metabolism may hamper bone formation [44, 45]. Lipolysis of adipocyte lipid droplets may release fatty acids such as palmitate, ultimately interfering with the differentiation and function of osteoblasts by disrupting β-catenin and Runx2 signalling mechanisms [18, 19]. In addition, the accumulation of free fatty acids may have lipotoxic effects on osteoblasts by inducing autophagic and apoptotic mechanisms, leading to long-term bone loss [46].
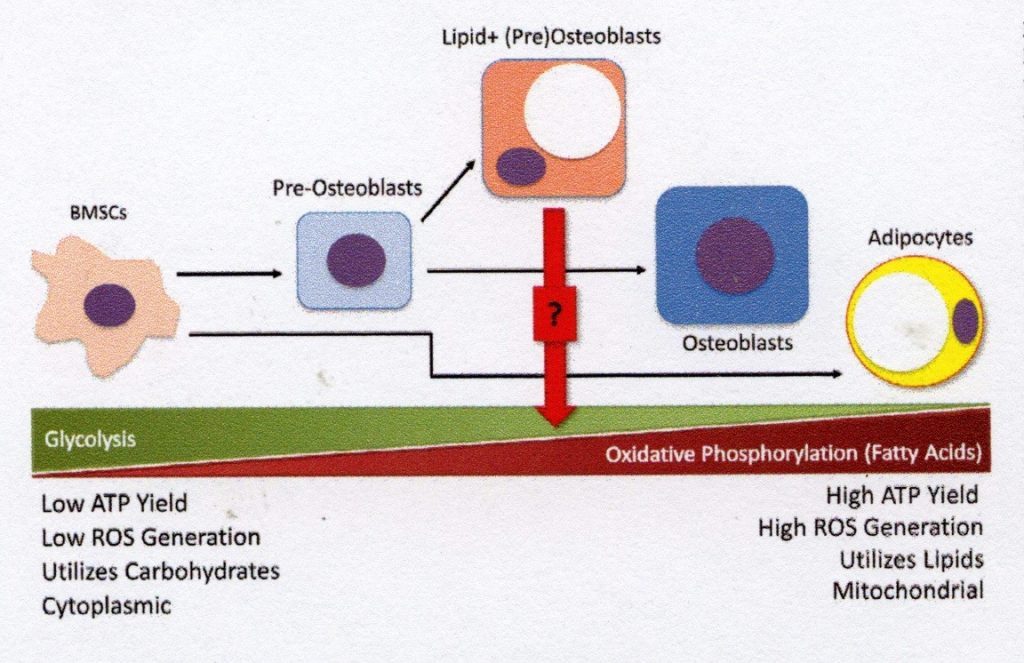
- The identity of the adipocyte osteoblast
Intracellular lipid deposition in osteoblasts raises concerns regarding their identification of marrow adipocytes, as well as the purpose of lipid storage in bone. Although the molecular identity of lipid-storing, non-adipogenic cells in bone marrow is still at an early stage, it is speculated that these cells may represent an intermediate stage between osteogenic and adipogenic differentiation endpoints. Since BMSCs are the common precursors of osteoblasts and adipocytes, the overlap in their morphologies indicates a potential for transdifferentiation between cell types (rather than a terminal differentiation) under certain physiological conditions [47].
6. Summary and future directions
…BMSC commitment to osteogenesis is an important pathway during embryogenesis, skeletal growth and bone remodelling to ensure correct tissue function. The generation of osteoblasts from BMSCs depends on strictly regulated signalling mechanisms (e.g. Wnt/β-catenin signalling), hormonal factors (e.g. PTH, glucocorticoids), epigenetic regulators (e.g. Hdac3) and the activity of specific transcription factors such as Runx2 and Osxl. BMSC-derived osteoblasts express genes and proteins associated with the production of osteoids (which convert to a calcified bone matrix), and this energy-intensive process tends to shift the energetic profile of these cells from a predominantly glycolytic profile to one which is more complex and which includes oxidative phosphorylation.
There is increasing evidence that differentiation of BMSCs into osteogenic, adipogenic and chondrogenic populations requires intermediate steps, and that transition cell types may exist. What is interesting for the purposes of this article is the accumulation of lipid droplets in non-adipogenic cell types – a phenomenon which occurs in various soft tissues throughout the body during ageing and in certain pathologies. One argument for increased bone marrow lipid storage is that cells that are positive for osteogenic markers are able to use lipid droplets as energy stores under energetically challenging conditions. A further plausible argument for the deposition of osteogenic lipids is that adipogenesis is the standard commitment pattern of BMSCs. As such, epigenetic regulators and signalling mechanisms produced by the microenvironment of the bone marrow may downregulate adipogenesis in favour of bone formation and its loss of function. Regulators (for example, due to ageing, stress or pathological conditions) may trigger reversal of osteogenic binding. This ‘de-differentiation’ step might be an intermediate step in changing the line that may favour adipogenesis, but current techniques for tracing the cells in question limit these research efforts. An emerging school of thought in bone marrow fat research suggests that osteoblasts and adipocytes may undergo the phenomenon known as transdifferentiation, or a change between the morphologies of these terminal cell types, rather than an intermediate differentiation step. Despite recent evidence, it is still difficult to confirm whether observations of transdifferentiation are real or due to the conditions under which BMSCs are cultured, as the available substrates and fuel sources in culture media may influence the binding of these cells to particular lineages.